Main Content
Regulation of skeletal muscle cell plasticity in health and disease
Skeletal muscle has an enormous capacity to adapt to external stimuli including physical activity, oxygen levels, ambient temperature, nutrient availability and composition. When these processes are dysregulated, the risk for many chronic, highly prevalent diseases is increased, e.g. obesity, type 2 diabetes, cardiovascular disorders, osteoporosis, neurodegenerative events, mood disorders, age-related muscle wasting (sarcopenia), and certain cancers. Moreover, sporadic or hereditary muscular dystrophies are likewise caused by abnormalities in skeletal muscle. Surprisingly, despite the high clinical relevance, the underlying molecular causes for many of these pathologies is unknown, and accordingly, treatment options are rare or non-existent. Curiously, regular exercise training is an excellent mediator of prevention and therapy for many of these pathologies, ultimately improving life quality and expectancy. Nevertheless, the complexities of muscle plasticity in single exercise bouts or prolonged training are only rudimentarily understood, both for endurance- as well as resistance-type of activities. Therefore, a better understanding of the molecular underpinnings of skeletal muscle cell biology, function and plasticity in health and disease on different levels and scales is clearly needed. In our group, we aim at linking molecular mechanisms to physiological and pathophysiological processes in skeletal muscle, using a multipronged, broad and state-of-the-art methodological approach (Figure 1). Our projects span the range from computational analysis and modeling to molecular biology and biochemistry, cell culture studies in cell lines and primary cells, and ultimately mouse models with the help of extensive phenotyping capabilities for longitudinal studies (including different transgenic and disease models, nutritional/dietary and exercise interventions, aging and other experimental paradigms). Moreover, we make use of the cutting-edge core facilities of the Biozentrum, and have numerous collaborations with other groups in-house as well as at other institutes in Switzerland and abroad. Finally, we validate and expand our findings in the human context together with exercise physiologists and clinicians.
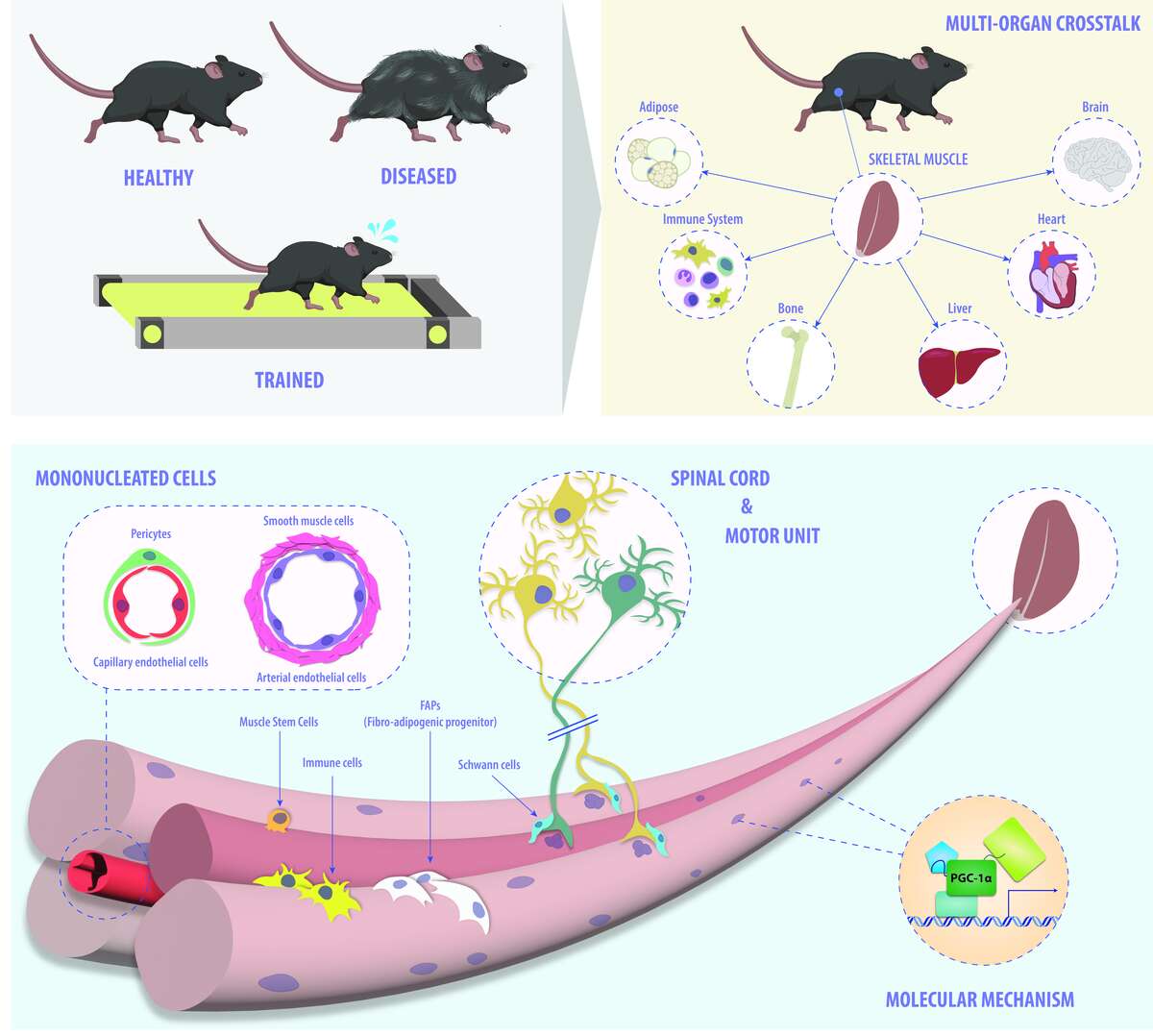
Figure 1. Skeletal muscle plasticity across different scales. Our group is interested in identifying the molecular underpinnings that control physiological and pathophysiological processes in skeletal muscle: from mechanistic aspects of transcriptional regulation to multicellular interactions within muscle tissue as well as crosstalk between tissues in the context of physiological and pathological events in mice.
Our studies of muscle plasticity across different scales are centered on the regulation of complex, spatio-temporally controlled transcriptional networks in the different nuclei of the syncytial myofiber. We aim at identifying the local environment that controls the engagement of various signaling pathways, leading to the assembly of transcriptional hubs in myonuclei, for example those formed by the transcriptional coregulator and RNA-binding protein peroxisome proliferator-activated receptor γ coactorator 1α (PGC-1α) in liquid-liquid phase separated condensates encompassing multiprotein transcriptional complexes and regulatory DNA elements. The interrogation of the ensuing transcription, informed by epigenetic modifications, reveals the adaptation of spatially separated myonuclei over a temporal scale, e.g. during contraction, recovery and regeneration after exercise. In addition, these processes are studied in the pathological context, e.g. insulin resistance and type 2 diabetes, sarcopenia, cancer cachexia, or muscular dystrophies.
Second, we are interested in the multicellular cross-talk within muscle tissue. Single cell and single nucleus RNA sequencing (scRNAseq and snRNAseq) experiments revealed a multitude of different cell types, many of which change dynamically either in number or activation after physiological or pathophysiological perturbations (Figure 2). The multi-directional cross-talk and signaling thereby is crucial to maintain tissue homeostasis and induce plastic changes. As an example, we are interested in the regulation and function of the neuromuscular junction (NMJ), the motor unit and accessory cells, including the neuronal circuitry important for motor unit activity, e.g. the proprioceptive and vestibular inputs.
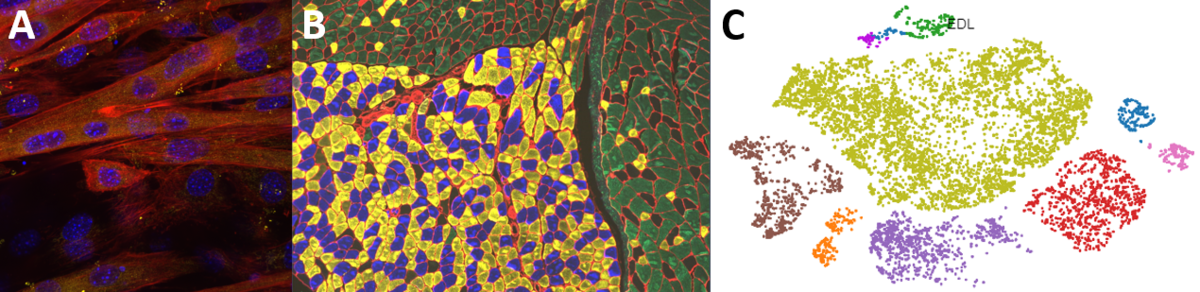
Figure 2. Multicellular dynamics in skeletal muscle. (A) Differentiated muscle cells in culture (actin filaments in red, cell nuclei in blue). (B) Skeletal muscle fiber type determination (type I fibers in blue, type IIa fibers in yellow, type IIx fibers in black, type IIb fibers in green, cell membranes in red). (C) single cell sequencing (scRNAseq) results of the extensor digitorum longus (EDL) muscle. Cell population clusters are marked by different colors.
Finally, we study the systemic consequences of muscle (dys-)function by assessing the adaptations in other organs, and by pinpointing the relevant auto-, para- and endocrine mediators such as myokines or metabokines. On one hand, the molecular mechanisms that are responsible for exerting and coordinating the broad effect of exercise training on different tissues are thereby revealed. On the other hand, the inter-organ crosstalk that contributes to pathological events, e.g. between tumor cells and skeletal muscle, is elucidated. Collectively, a comprehensive overview on the systemic interactions between skeletal muscle and other organs is thereby obtained, allowing an unraveling of the processes that contribute to such complex phenotypes (Figure 3).
For all of these projects, we use a two-pronged approach: the study of endurance and resistance exercise helps to reveal how these systems are engaged in the physiological setting representing the main biological function of skeletal muscle (fiber contraction). Juxtaposed to this context, we use different genetic models, diets and other interventions to interrogate the pathological consequence of inadequate muscle activity of aberrant muscle functionality. Hence, to gain a broad understanding of the pathological context, we are interested in inactivity and the related muscle atrophy, sarcopenia and the aging process, cancer cachexia, and muscular dystrophies. Intriguingly, many of the pathways that are engaged in contracting muscle in exercise are mirrored in muscle diseases, and therefore, the combined knowledge of physiology and pathophysiology helps to identify relevant signaling pathways, transcriptional processes and other molecular events that are important for muscle function, are affected in diseases, and thus could potentially be leveraged for novel therapeutic approaches. The human relevance of our results is validated and investigated in collaboration with exercise physiologists, clinicians, biotech and pharmaceutical companies.