Main Content
Motor circuit function
Behavior is the common output of the nervous system. Despite their importance, the mechanisms by which neuronal circuits produce actions appropriately aligned with needs and intentions are poorly understood. To decipher how motor circuits engage in the accurate and timely control of movement and the generation of diverse actions, we unravel neuronal subpopulations and study their function in the execution and learning of motor programs.
The motor system consists of a hierarchy of circuit modules, with three main components that contribute to movement control: (1) centers in the brain and brainstem involved in the selection of appropriate motor plans that provide essential regulatory signals for execution to the spinal cord; (2) circuits in the spinal cord responsible for motor plan execution and the generation of basic patterns of motor activity according to supraspinal commands; (3) sensory feedback circuits that inform the nervous system about past action and perturbations. We dissect these circuit elements from various angles to understand how precisely connected neuronal circuits lead to diversification of body action according to motor plans.
We use multi-facetted approaches combining many technologies, including state-of-the-art mouse genetics, the development and implementation of viral technologies for transsynaptic circuit tracing and functional manipulation by opto- and pharmacogenetics, quantitative behavioral analysis, and in vivo electrophysiology. These approaches allow us to assess connectivity and manipulate function to determine the role of defined circuits in animal behavior. They also enable us to uncover mechanisms involved in motor circuit assembly and function, as well as circuit plasticity during motor learning and response to disease or injury.
Discovery of brainstem circuits involved in different motor behaviors
Natural movement depends on the function of descending pathways from supraspinal centers to the spinal cord. This is strikingly obvious in patients with complete spinal cord injury, who are unable to move muscles controlled by spinal segments below the lesion. Classical studies provided evidence that the brainstem is involved in the control of a variety of movements. Yet, we still lack a deep understanding of the organization and connectivity of identified neuronal subpopulations in the brainstem, how they intersect with executive circuits in the spinal cord, and how they function. We recently investigated the organization of the bidirectional connectivity matrix between the brainstem and spinal circuits (Esposito et al., 2014; Pivetta et al., 2014).
Our experiments have revealed highly specific connections between different brainstem nuclei and spinal neurons (Fig.?1), providing insight into the sophisticated interactions that carry motor control commands from the brainstem to the spinal cord (Esposito et al., 2014). In behavioral experiments, we began to address the functional implications of this striking connectivity matrix, focusing on the medullary reticular formation ventral part (MdV), a brainstem nucleus with highly preferential connections to forelimb motor neurons (Fig.?1). Selective ablation or silencing of glutamatergic MdV neurons showed them to be specifically required for skilled motor tasks, in particular for the grasping phase of the single food pellet retrieval task involving forelimbs.
These findings support a model in which distinct brainstem subpopulations control aspects of motor behavior through specific targeted spinal subcircuits. More generally, they provide important insight into circuit-level mechanisms essential for task-specific motor subroutines during the execution of complex motor programs. They also raised the question of the general applicability of the concept of anatomical subdivision of brainstem neurons according to functional criteria, a theme we have further exploited in work described below.
Brainstem circuits for locomotor speed control
Animals and humans use locomotion as a means to get from one place to another. Despite their importance, the neuronal circuits regulating locomotion are far from understood. An especially fascinating subject is the identification of speed-regulatory components in the nervous system, given that animals regulate speed instantaneously when adjusting to changing situations: from casual walking, to high-speed running, to stopping. Our initial anatomical discovery project for neuronal populations in the brainstem with access to the spinal cord was the starting point for this project (Esposito et al., 2014). We focused on brainstem regions in which neurons exhibit equal anatomical access to neuronal circuits in the cervical and lumbar spinal cord, the spinal segments in charge of regulating fore- and hindlimbs, respectively. We reasoned that instructions for full-body regulatory parameters during locomotion should be equally distributed throughout the spinal cord.
A highly interesting region in this context resides in the caudal brainstem (Capelli et al., 2017). We found that neuronal subpopulations in this region with different neurotransmitter identities and spinal projections are intermingled and located in four adjacent domains. Despite the presence of many spinally projecting neurons, optogenetic activation of these regions as a whole did not influence locomotion. Only after dissection of these neurons genetically into excitatory and inhibitory populations could functional roles be assigned. Most strikingly, optogenetic activation of glutamatergic neurons in the small caudal brainstem subregion Lateral Paragigantocellular nucleus (LPGi) induced short-latency locomotion in stationary animals and speeding-up in animals already moving. Stimulation of glutamatergic neurons in neighboring subdomains did not induce locomotion. In sharp contrast to the results for excitatory neurons, stimulation of intermingled glycinergic neurons induced behavioral arrest; different forms of arrest from simple stopping to full-body collapse were observed, depending on the region stimulated. Interestingly, excitatory and inhibitory LPGi neurons target essentially complementary regions within the spinal cord, pointing to a possible circuit mechanism by which descending pathways of different brainstem populations can elicit opposing body behaviors.
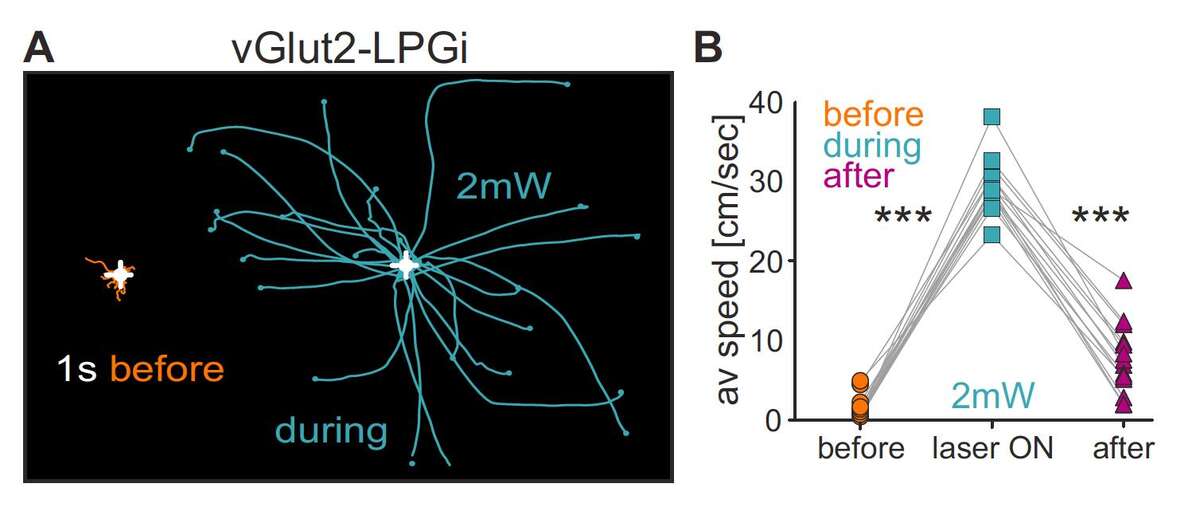
Fig. 2. Speed control circuits in the caudal brainstem. A. Effect of optogenetic stimulation of glutamatergic (vGlut2) LPGi neurons on locomotion. Behavioral trajectories of a mouse 1 s before (orange) and during (cyan) optogenetic stimulation at 2-mW stimulation intensity. B. Analysis for many trials of average speed values during these time periods and 1 s after (purple). Adapted from Capelli et al., 2017
Important open questions based on these findings were the role of locomotion-inducing glutamatergic LPGi neurons during natural behavior and how they are regulated by upstream inputs. We found that glutamatergic LPGi neurons are essential for high-speed locomotion and that they receive input from many upstream regions in the motor system. Input from the midbrain locomotor region MLR was particularly interesting, since it could positively tune locomotor speed through excitatory LPGi neurons. Thus, neurons in the midbrain signal magnitude of locomotor speed through specific populations of caudal brainstem neurons.
In summary, behaviorally opposing locomotor functions in the caudal brainstem were masked previously by the unexposed diversity of intermingled neuronal subpopulations. Together with our previous work on neuronal populations in the brainstem, the results highlight an amazing and previously unrecognized degree of synaptic specificity for descending motor control pathways. They suggest the existence of dedicated hubs in the brainstem for action program selection, represented by specific neuronal subpopulations.
Organization and function of motor circuits in the spinal cord
Neurons in the spinal cord play fundamental roles in the execution of motor programs. Their specific wiring is essential for the implementation of instructive signals from the brain arriving in the spinal cord. In recent work, we have uncovered extensive and precisely organized long-distance communication circuits involved in interlimb coordination (Ruder et al., 2016) and demonstrated that subtle miswiring of genetically-identified specific neuronal components in the spinal cord can lead to behavioral alternations (Satoh et al., 2016). Together, the two studies highlight the importance of wiring specificity in the spinal cord for motor program execution.
More specifically, we employed mouse genetics and viral approaches to define the organizational principles of long-distance spinal projection neurons (Ruder et al., 2016). Based on neurotransmitter identity, developmental origin, and projection pattern, we found that spinal segments assigned to fore- or hindlimb regulation are bidirectionally coupled through synaptic connections by specific populations of spinal neurons. Our work further demonstrated that selective ablation of descending spinal neurons connecting cervical and lumbar segments results in defects in whole-body movement parameters, including postural stability during exploration and interlimb coordination deficiencies at high speeds.